Age-related cataract, also referred to as senile cataract, is the most common form of cataract. It primarily affects middle-aged and elderly individuals, typically those over 50 years of age, with a significantly increasing prevalence as age advances.
The condition represents degenerative changes in the lens due to aging and results from the combined effects of multiple factors. Risk factors include age, occupation, sex, ultraviolet radiation exposure, diabetes, hypertension, and malnutrition.
Clinical Manifestations
Age-related cataracts often affect both eyes, but the onset may occur at different times, and the severity may vary between the two eyes.
Symptoms
Decreased Visual Acuity
This is the most prominent and significant symptom. Mild opacities on the peripheral lens may not affect vision, but even small or mild opacities in the central lens can lead to significant vision impairment. Vision may paradoxically worsen in bright light due to pupil constriction, which reduces the light entering the eye, while appearing better in dim light. Severe lens opacities can reduce vision to mere light perception.
Reduced Contrast Sensitivity
Patients experience a marked decline in contrast sensitivity, particularly in high spatial frequencies.
Refractive Changes
Nuclear cataracts increase the refractive index of the lens nucleus, enhancing lens power and causing myopia (also known as "second sight" in presbyopic patients). Pre-existing presbyopia may temporarily improve. Uneven opacities in the lens can also lead to lenticular astigmatism.
Monocular Diplopia or Polyopia
Irregular lens opacities or water clefts can result in uneven refractive power across the lens, acting like a prism and causing monocular double or multiple images.
Glare
This symptom arises from light scattering caused by lens opacities.
Color Vision Changes
Enhanced absorption of blue-wavelength light by the cloudy lens results in reduced sensitivity to shorter wavelengths. Changes in the lens nucleus coloration may similarly alter color perception.
Visual Field Defects
Lens opacities can lead to varying degrees of visual field impairment.
Signs
Lens opacities can be observed and assessed through the naked eye, focused light, or slit-lamp biomicroscopy. Different types of cataracts exhibit distinct opacity patterns. Peripheral lens opacities may only be visible after pupil dilation.
Classification
Based on the location where the lens opacity begins, age-related cataracts are divided into three types: cortical, nuclear, and posterior subcapsular cataracts.
Cortical Cataract
This is the most common type of age-related cataract. It progresses through four stages:
Incipient Stage (Early Stage)
When observed through slit-lamp biomicroscopy, vacuoles and water clefts become visible in the lens cortex. These clefts expand from the periphery to the center, forming wedge-shaped opacities in the anterior and posterior cortex, often with feather-like edges pointing to the center. These wedge-shaped opacities may meet at the equatorial region, forming radial opacities. Upon pupil dilation, ophthalmoscopy may reveal spoke-like or patchy shadows against a red reflex. Peripheral opacities in the early stage may not significantly impair vision, and progression is typically gradual over several years.
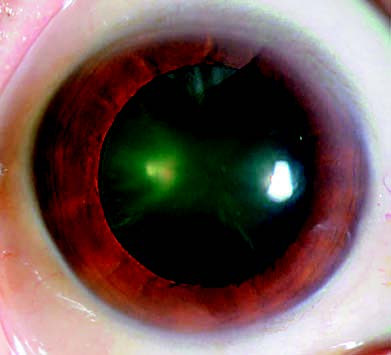
Figure 1 Incipient-stage cataract
Wedge-shaped opacities are observed in the peripheral lens.
Intumescent (Swollen) or Immature Stage
Lens opacities become more pronounced, and changes in osmotic pressure cause fluid accumulation, leading to cortical swelling and an increase in lens volume. This results in anterior chamber shallowing, which, in individuals predisposed to angle-closure glaucoma, can trigger acute glaucoma attacks. The lens appears grayish-white, and with oblique illumination, the iris casts a crescent-shaped shadow on the cortical opacity, a characteristic feature of this stage referred to as an "iris projection." Visual acuity significantly declines, and fundus examination becomes difficult.
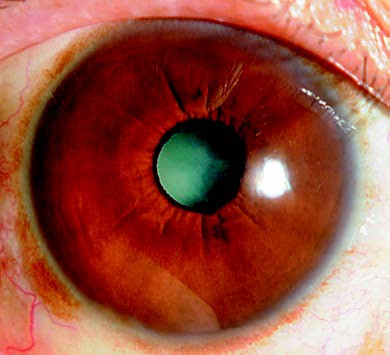
Figure 2 Intumescent-stage cataract
A crescent-shaped shadow is noticeable on the iris.
Mature Stage
The lens loses water content, swelling subsides, and lens volume decreases, causing the anterior chamber depth to normalize. The lens becomes entirely opaque and appears milky-white, sometimes with visible calcium deposits on the capsule. Vision decreases to hand motion or light perception levels, and the fundus is no longer visible.
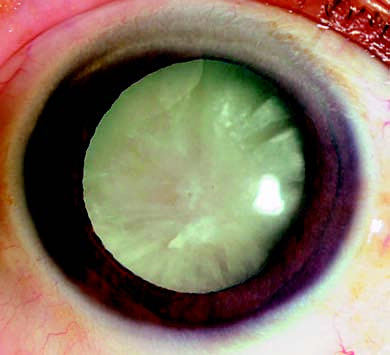
Figure 3 Mature-stage cataract
The lens appears completely opaque with a milky white coloration.
Hypermature Stage
If the mature stage persists for an extended period, over several years, the lens continues to lose water, resulting in a reduction in lens volume and wrinkling of the capsule, along with the formation of irregular white spots and cholesterol crystals. The anterior chamber deepens, and iridodonesis (iris trembling) may occur. The lens fibers undergo liquefaction and decomposition, appearing milky white. The brownish-yellow lens nucleus sinks within the capsule and may move with changes in body position, a condition referred to as Morgagnian cataract. In some instances, vision may suddenly improve after the lens nucleus sinks.
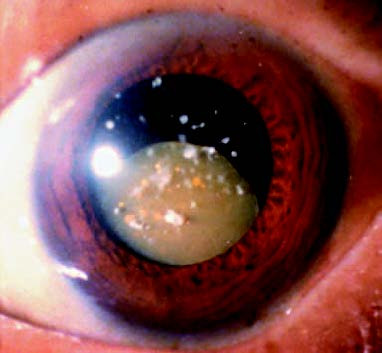
Figure 4 Hypermature-stage cataract
The lens nucleus sinks.
In the hypermature stage, the lens capsule undergoes degeneration and becomes increasingly permeable or develops microfractures, resulting in the leakage of liquefied cortical matter outside the lens capsule. This can lead to uveitis triggered by the lens proteins. Cortex material that remains in the aqueous humor for an extended period can accumulate in the anterior chamber angle or be phagocytosed by macrophages, potentially blocking the angle and causing phacolytic glaucoma. Degeneration of the suspensory ligament increases the risk of lens dislocation or subluxation, and rupture of the capsule may result in the nucleus becoming dislodged. If the dislodged lens or nucleus obstructs the pupillary area, it can lead to secondary glaucoma. Uveitis and glaucoma caused by these complications necessitate urgent surgical intervention.
Nuclear Cataract
This type of cataract develops earlier and progresses slowly. Opacity begins in the fetal or adult nucleus of the lens. In the early stages, the nucleus appears yellow and may not be easily distinguishable from normal nuclear sclerosis. Nuclear sclerosis, a physiological process, involves a gradual increase in the density and color of the lens nucleus with age due to lifelong lens growth, without significantly affecting vision.
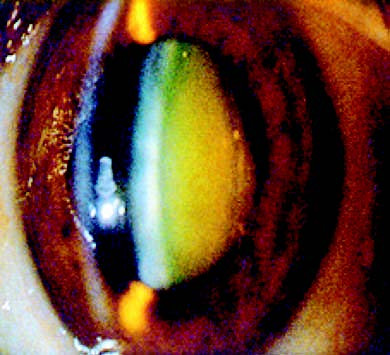
Figure 5 Nuclear cataract
The lens nucleus exhibits a yellowish-brown opacity.
As nuclear cataract progresses, the color of the nucleus darkens, transitioning through yellowish-brown, brown, and brownish-black, and finally reaching black in some cases. Early on, the increased refractive power of the nucleus can lead to myopia, causing a gradual reduction in distance vision while improving near vision. In advanced stages, severe nuclear opacities may prevent examination of the fundus, leading to profound vision loss.
Posterior Subcapsular Cataract
Posterior subcapsular cataracts involve brownish-yellow opacities in the superficial posterior cortex of the lens. These consist of a dense cluster of small dots, along with vacuoles and crystal-like particles, giving an appearance similar to scorched rice. Due to the central location of the opacity on the visual axis, vision impairment becomes noticeable even in the early stages.
Posterior subcapsular cataracts progress slowly and may eventually involve cortical and nuclear opacities, culminating in a complete cataract.
Description and Classification of Lens Opacities
The Lens Opacities Classification System (LOCS) is used to evaluate the extent and severity of lens opacities. It provides a straightforward approach for use in cataract studies, epidemiological surveys, and the assessment of drug efficacy.
The LOCS II classification system was widely used for many years. Under this system, the pupil is fully dilated and the lens is examined using slit-lamp microscopy and retroillumination. Nuclear opacities (N), cortical opacities (C), and posterior subcapsular opacities (P) are graded on a scale from N0 to N3, C0 to C5, and P0 to P4, respectively.
In recent years, the LOCS III classification system, which offers finer granularity, has gained acceptance. LOCS III employs a set of standard color slit-lamp and retroillumination photographs to classify nuclear opacities (NO), cortical opacities (C), posterior subcapsular opacities (P), and nuclear color (NC) into standard grades ranging from NO1 to NO6, C1 to C5, P1 to P5, and NC1 to NC6. Patient images taken after pupil dilation can be compared with the standard photographs to assess the severity of cataracts. Intermediate levels of opacities or color between two grades are denoted using decimal values.
Grading of Lens Nucleus Hardness
Accurate assessment of lens nucleus hardness is crucial for determining the indications and techniques for phacoemulsification. Clinically, grading is often based on the color of the nucleus, with the Emery-Little nuclear hardness grading system being one of the most commonly used methods. This system classifies nuclear hardness into the following five grades:
- Grade I: Transparent lens, no nucleus, soft.
- Grade II: Yellow-white or yellow nucleus, soft.
- Grade III: Deep yellow nucleus, medium hardness.
- Grade IV: Brown or amber-colored nucleus, hard.
- Grade V: Brownish-black or black nucleus, extremely hard.
Diagnosis
Dilated fundoscopic or slit-lamp biomicroscopic examination of the lens is required to confirm diagnosis. Diagnosis is based on the morphology of lens opacities and associated visual impairment. If vision loss does not correlate with lens opacities, further investigations are necessary to identify other potential causes of vision decline, thus preventing misdiagnosis of other ocular conditions as cataracts.
Treatment
Pharmacological Treatment
Extensive research has been conducted over the years on the etiology and mechanisms underlying cataracts, with various medications being developed to target their presumed causes. Despite the availability of over a dozen anti-cataract drugs, including traditional Chinese medicines, their clinical efficacy remains uncertain.
Surgical Treatment
Surgery remains the primary and most effective treatment for all types of cataracts. Common surgical methods include phacoemulsification under a surgical microscope, femtosecond laser-assisted cataract surgery, or extracapsular cataract extraction combined with intraocular lens implantation, all of which can deliver satisfactory outcomes.
Indications for Surgery
The primary indication is when cataract-related functional vision loss significantly impairs a patient's ability to perform daily tasks, and surgery offers the potential to improve vision.
Lens extraction is suitable in cases where opacity obstructs the diagnosis or treatment of posterior segment conditions, such as retinal detachment, diabetic retinopathy, or endophthalmitis.
Surgery may be indicated in the presence of clinically significant anisometropia combined with cataracts.
Cataract surgery can also be considered for lens-induced conditions, such as phacolytic inflammation, phacoanaphylactic reactions, or angle-closure glaucoma triggered by an intumescent lens.
Even in eyes with no remaining visual potential, mature or hypermature cataracts that turn the pupillary area white and affect cosmetic appearance could be treated surgically upon patient request.
Contraindications for Surgery
Surgery is not recommended when the patient is unwilling to proceed, or when informed consent from the patient or their legal proxy cannot be obtained.
Surgery may not be warranted if the patient’s quality of life is unaffected, or if their visual needs can be met with corrective lenses or other assistive devices.
Surgery is not advised when no improvement in vision is anticipated postoperatively, and there are no other indications for lens removal.
Severe systemic conditions that compromise the safety of surgical procedures also constitute contraindications.
Preoperative Examinations and Preparations
Ophthalmic examinations include evaluation of visual acuity, light perception and projection, and red-green color discrimination.
Examination using slit-lamp microscopy and ophthalmoscopy is conducted to assess the condition of the cornea, iris, anterior chamber, retina, and lens opacities while ruling out active ophthalmic inflammation.
Specialized investigations include:
- Measurement of intraocular pressure (IOP).
- Assessment of corneal curvature and axial length to calculate the appropriate intraocular lens power.
- Examination of corneal endothelial cell density.
- Ocular B-scan ultrasound imaging.
Systemic examinations include:
- Control of blood pressure and blood glucose levels in patients with hypertension or diabetes.
- Comprehensive assessment of cardiac, pulmonary, hepatic, and renal functions to ensure surgical tolerance, with internal medicine consultations as needed.
Postoperative visual prognosis:
- Light Projection Test: A simple and effective method to evaluate retinal function. An inaccurate light projection test may indicate impaired retinal function.
- Visual Electrophysiology Tests: These include electroretinogram (ERG) and visual evoked potential (VEP) studies. ERG assesses the functionality of retinal photoreceptor cells (rods and cones), while VEP reflects abnormalities in macular activity and optic nerve function.
- Laser Interferometry: Laser interferometry produces two-dimensional monochromatic interference fringes on the retina through cataractous lenses. The patient's ability to resolve such fringes correlates closely with macular visual function, providing insights into potential postoperative outcomes.
Preoperative preparations include procedures such as conjunctival sac and tear duct irrigation to reduce infection risks, along with the application of mydriatics to dilate the pupil.
Surgical Methods
Cataract surgical techniques have advanced rapidly over the last two centuries. In particular, the development and application of microsurgical techniques and intraocular lens implantation over recent decades have led to a qualitative leap in cataract surgery, making it one of the most dynamic and rapidly evolving fields in modern ophthalmology.
Couching of the Lens
This technique involves mechanically severing the zonular fibers to dislocate the cloudy lens into the vitreous cavity. Due to the high rate of postoperative complications, this method has been largely abandoned.
Intracapsular Cataract Extraction (ICCE)
This procedure involves the complete removal of the cloudy lens, including the capsule. While the technique is straightforward, it requires a large incision and is associated with a high risk of complications. As a result, ICCE is rarely performed in modern clinical practice.
Extracapsular Cataract Extraction (ECCE)
This technique removes the opaque lens nucleus and cortical material while preserving the posterior capsule. ECCE is performed under a surgical microscope and requires advanced surgical skills. Preservation of the posterior capsule minimizes disruption to and damage of intraocular structures, reducing complications such as vitreous loss. It also facilitates the smooth implantation of a posterior chamber intraocular lens.
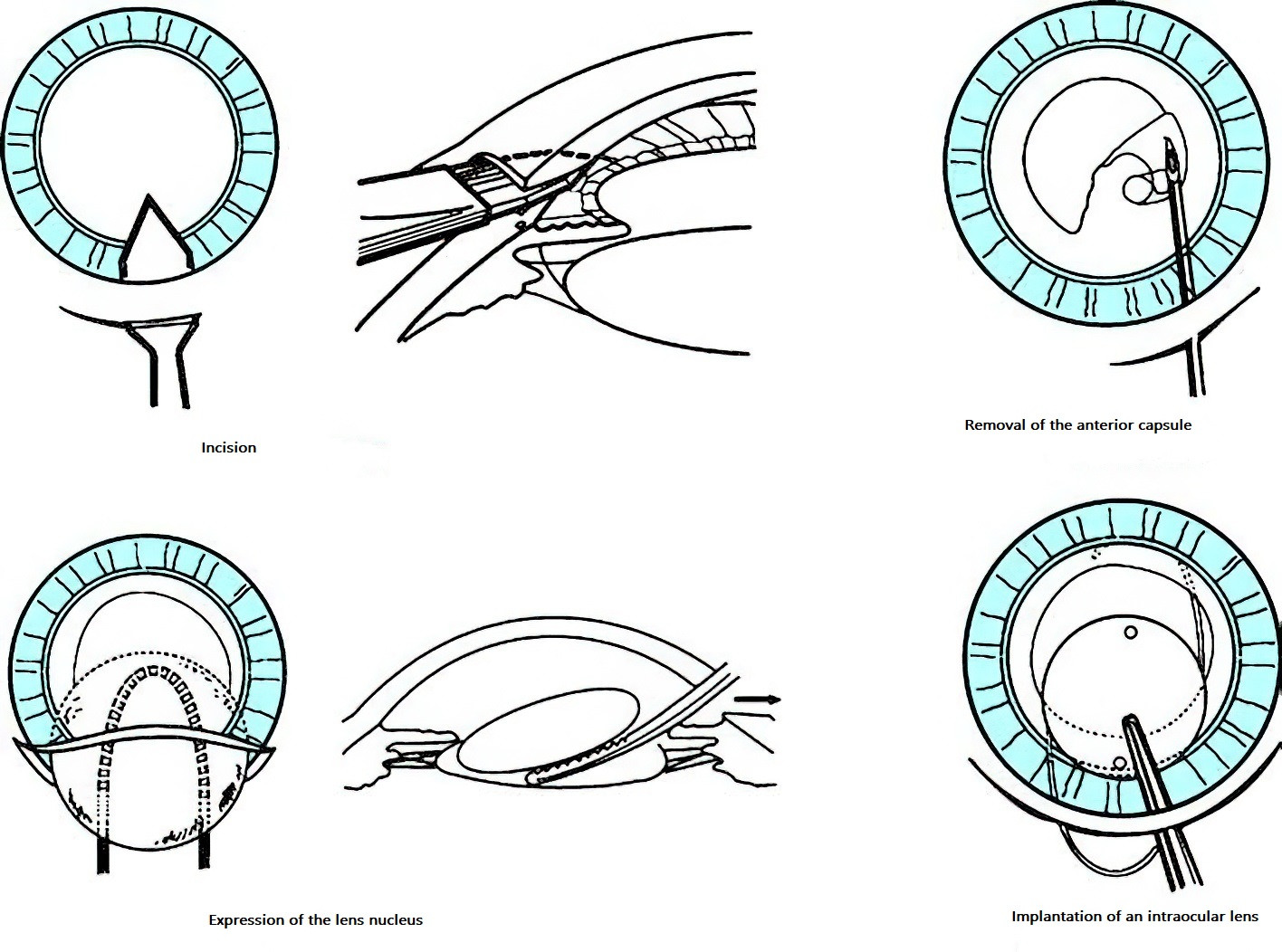
Figure 6 Schematic diagram of extracapsular cataract extraction (ECCE)
Ultrasonic Phacoemulsification
This method uses ultrasonic energy to emulsify the lens nucleus and cortex, which are then aspirated while preserving the posterior capsule. Introduced in the 1960s, phacoemulsification has seen rapid advancements and, combined with the use of foldable intraocular lenses, has become a mature and widely used technique. Phacoemulsification reduces the surgical incision to 3 mm or smaller, offering advantages such as minimal tissue damage, no need for sutures, shorter surgical times, faster visual recovery, and reduced corneal astigmatism. The procedure can also be performed under topical anesthesia.
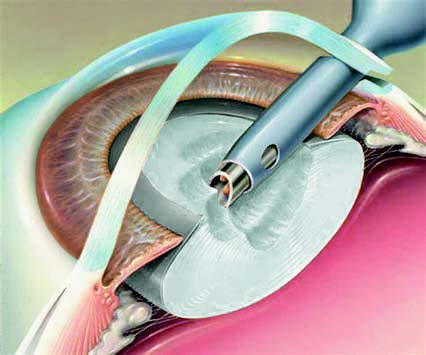
Figure 7 Schematic diagram of ultrasonic phacoemulsification
Micro-incision phacoemulsification has further reduced incision sizes to 1.5–2.2 mm, significantly minimizing tissue trauma and postoperative corneal astigmatism, while allowing for even faster visual recovery.
Femtosecond Laser-Assisted Cataract Surgery (FLACS)
The femtosecond laser operates using ultrashort laser pulses, offering advantages such as high instantaneous power, minimal focal spot size, strong penetrating ability, and exceptional precision. As a groundbreaking technology in cataract surgery over the past decade, it represents an advanced approach similar to robotic-assisted surgical systems.
The femtosecond laser can be used for capsulotomy, nucleus prefragmentation, and creation of corneal incisions, enhancing surgical precision, reducing tissue trauma, and improving the overall safety of the procedure. This technique has proven particularly beneficial for complex cases, such as hard nucleus cataracts, mature or hypermature cataracts, cataracts with shallow anterior chambers, low endothelial cell counts or corneal endothelial dystrophy, and axial high myopia. The use of the femtosecond laser has also contributed to a higher success rate and fewer complications in such cases.
Intraocular Lens (IOL) Implantation
Intraocular lenses provide the most effective method for refractive correction in aphakic eyes and are now widely utilized. IOLs are made from polymer materials that exhibit excellent optical properties and biocompatibility, allowing for rapid recovery of visual function, binocular single vision, and stereopsis after implantation.
Currently, the standard approach involves implanting foldable IOLs in the posterior chamber. As cataract surgery enters the refractive era, traditional monofocal IOLs may no longer meet the growing demand of patients for precise postoperative uncorrected vision at distance, intermediate, and near. As a result, presbyopia-correcting IOLs were developed and have evolved significantly over time, transitioning from early ring-shaped refractive designs to later ring-shaped diffractive designs, accommodating designs, zonal refractive designs, and alternating diffractive-refractive designs.
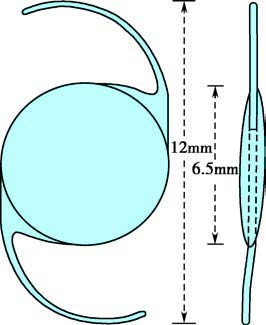
Figure 8 Monofocal intraocular lens
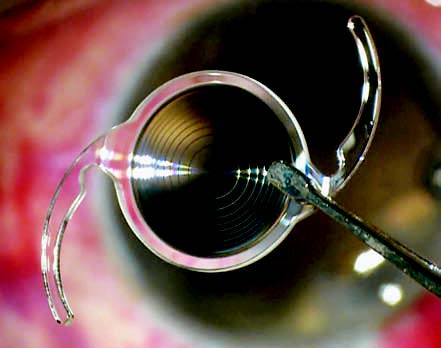
Figure 9 Bifocal intraocular lens
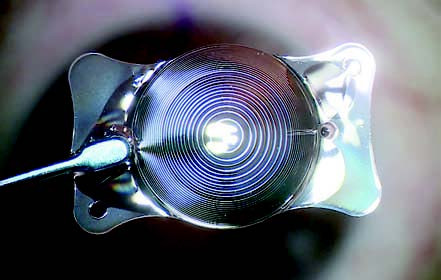
Figure 10 Trifocal toric intraocular lens
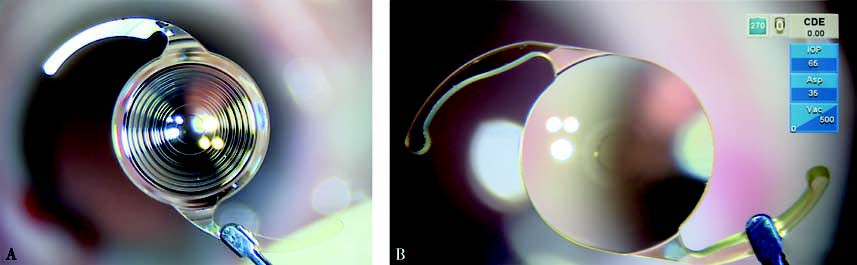
Figure 11 Extended depth-of-focus intraocular lens
A. Diffractive type
B. Refractive type
Today, presbyopia-correcting IOLs can be categorized based on their optical design into bifocal IOLs, trifocal IOLs, extended depth-of-focus IOLs (diffractive or refractive), and accommodating IOLs. Furthermore, ongoing research and clinical trials are focused on the development of novel IOL designs to address diverse patient needs.